The move from 10G non-coherent, to 100G coherent wavelengths in all forms of subsea transmission is more or less complete, and has happened faster than analysts originally estimated – with the 40G data rate rapidly falling out of favour once 100G economics became clear. Those economics were not only driven by increased spectral efficiency, but associated technologies such as coherent super-channels have become key factors for network operators to achieve service differentiation in a market that has long been seen as a commodity.
The Coherent Technology Miracle
While the DWDM industry has an excellent track record of increasing capacity and data rates over time, the move from 2010 onwards to coherent transmission has delivered a quantum leap in capacity-reach products that will be difficult to improve on in the near future [1].
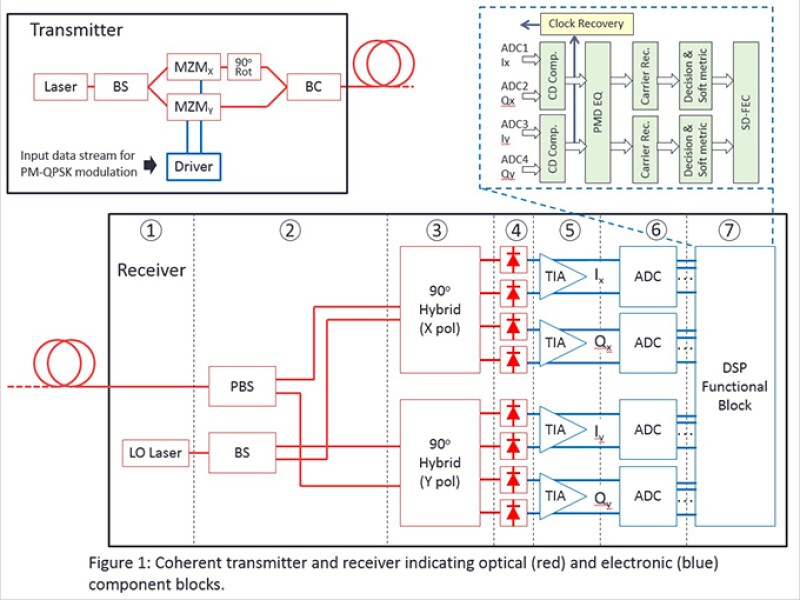
Figure 1 shows a typical 100Gb/s coherent optical circuit – with “first generation” coherent having all of its digital processing power in the receiver that was focussed on chromatic dispersion and polarisation mode dispersion (PMD) compensation; and “second generation” coherent adding transmitter-based digital pulse shaping, additional chromatic dispersion compensation, and receiver-based non-linear processing.
This optical circuit is extremely capable, delivering over a twenty-fold increase in the capacity-reach product compared to 10Gb/s intensity modulation with direct detection (IM-DD). A key point is that this achievement has been possible over existing submarine cable wet plant, enabling a massive upgrade capability as network operators replaced existing submarine line terminating equipment (SLTE) with coherent systems.
The next step, of course, is to take full advantage of new submarine cable types – in particular the large area/low loss G.654 fibre types that are being deployed in the latest subsea cable systems as positive dispersion cables (versus dispersion managed cable systems of previous deployment generations). These cables offer far better non-linear performance, coupled with significantly lower attenuation, to the point where it could be possible to close long Pacific spans using Pol Muxed QPSK modulation (instead of PMBPSK), and transatlantic cables using PM-8QAM, or perhaps even PM-16QAM modulation (instead of PM-QPSK). The value delivered by moving to these higher order modulation techniques is a dramatic increase in spectral efficiency, and thus a higher fibre capacity.
In March of this year, a major lab trial showed that it is possible to close transatlantic distances (in this case over 7,400km) with PM-8QAM over a LA/LL fibre provided by OFS. Following these successful lab trials likely that we will see field trials, and perhaps even real deployments of higher order modulation technologies on subsea cables in 2016.
Super-channels: Beyond 100Gbps
A number of academic papers have described how the industry is approaching a point of diminishing returns for capacity increases – and the conclusions are often simplistically represented as an approaching “capacity exhaust” in optical fibres. It may be true, for example, that the next generation optical cables spanning the Atlantic could be limited to between fifteen and twenty terabits per second total capacity, and a trans-Pacific next generation cable to between ten and twelve terabits per second. It may be possible to double these limits by simply adding L-Band transmission to the existing C-Band systems, but this would require very different wet plant from what is installed, or is being installed, today.
Thus, when the industry asks the question “what comes after 100Gbps” it is not typically a question of fibre capacity – this is a function of coherent technology, and the achievable fibre capacity is perfectly adequate for the current cycle of new deployments. Rather the question is focused on operational scalability; in other words, how can a single engineering team turn up significantly more subsea capacity in a given operational cycle? How can a network operator deliver services more quickly, and with more efficient cash flow?
The seemingly obvious answer is to simply crank up the symbol rate for the optical and electronic circuits shown in Figure 1. To deliver today’s typical 100Gbps data rate using PM-QPSK (which carries 4 bits per symbol) would imply a Baud rate between 32 and 35 GBaud (note that this quoted range of values is due to different sizes of Forward Error Correction, FEC, overhead used by different vendors). But increasing the Baud rate is not happening in practice for commercial products. The first single wavelength 100Gbps implementation, operating in 32-35GBaud range, began shipping almost five years ago, and here we are today with leading edge implementations still operating in the 32-35GBaud range. The reason is that the electronics that drives the optical circuit is now a bottleneck for serial processing. Chip power is increasing, but it is doing so by offering more parallel processing power.
There are two ways that next generation coherent implementation may overcome this limit, and thereby increase the data rate per line card. One is by moving to higher order modulation techniques; ie. PM-BPSK -> PM-3QAM -> PM-QPSK -> PM-8QAM -> PM-16QAM (which carry 2, 3, 4, 6 and 8 bits per symbol respectively). As pointed out above, moving along this scale increases spectral efficiency – with each modulation symbol “carrying more bits”. But at the same time the optical reach will drop, with PM-16QAM losing about 80% of the reach compared to PM-QPSK, for only double the line card or fibre capacity.
The other approach is to “go parallel” with multiple 100Gbps optical carriers. This means implementing multiple optical circuits, each operating at the optimum Baud rate to achieve a balance between capacity and reach, on a single line card so that all the capacity can be brought into service in a single operational cycle. As mentioned above, the resulting optical signal is called a “super-channel”, and it is the direction that the entire optical industry is now taking to achieve greater operational scalability. Super-channel subsea systems are now widely deployed in all parts of the world, from the longest Pacific routes, through to cables that circle South America and Africa, and crisscross South East Asia and the Mediterranean Sea.
Using today’s typical 500Gbps super-channel line cards, a single engineer can turn up five times as much capacity in the time it takes to bring a 100Gbps transponder into service; and the next generation of super-channel line cards will deliver 1.2Tbps of capacity (an ideal way to support, for example, the next generation of 400GbE services).